Development of a Detection System
For our system to work, we require a method to detect the presence of the A1 or A2 allele of CSN2. In our system, annealing of the spacer and flap oligos to the correct CSN2 allele, creates a Holiday-junction like structure, that is cleaved by the Flappase. In the Invader assay, the 5’ end of the Flap oligo is tagged with a fluorophore, and the 3’ end is tagged with a quencher. When cleaved, the flap and the fluorophore are released from quenching, yielding a fluorescent signal.
For a field detection system, we therefore require a robust fluorophore that can yield a strong signal. In addition, we require a field-compatible fluorometer that can be used to detect the signal from that fluorophore (Figure 1).

Figure 1: Overview of a detection system. In a reaction chamber cleavage of flap oligos yields a dequench fluorophore. Excitation of the fluorophore at the correct wavelength yields light at the emission wavelength, which can be detected.
Selection of a Fluorophore-quencher pair
To make this system work, we had to investigate which fluorophore and quencher pair to use. We considered the quantum yield of the fluorophore and the absorption and emission spectra of each potential tag and quencher. We aimed for a highly efficient fluorophore where the quencher’s absorbance matched the fluorophore’s emission. The properties of the fluorophore-quencher pairs that we investigated are given in the table below.
Table 1. Potential fluorophore and quencher pairs considered (1).
Quantum yield of fluorophore |
Fluorophore (absorbance max., |
Quencher (absorbance max., range in nm) |
0.92 |
Alexa Fluor. 488 (492,517) |
Iowa Black FQ (531,420-520) |
? |
Rhodamine green-X (504,531) |
Iowa Black FQ (531,420-520) |
0.9 |
ATTO 532 (534,554) |
Black Hole Quencher 1 (534,480-580) |
0.8 |
ATTO 550 (560,575) |
Black Hole Quencher 1 (534,480-580) |
0.92 |
ATTO 565 (570,591) |
Black Hole Quencher 2 (579, 560-670) |
0.8 |
ATTO Rho101 (592,609) |
Black Hole Quencher 2 (579, 560-670) |
0.79 |
Alex Fluor. 546 (556,571) |
Black Hole Quencher 1 (534,480-580) |
0.66 |
Alexa Fluor. 594 (584,616) |
Black Hole Quencher 2 (579), 560-670 |
0.61 |
Alexa Fluor. 532 (527,553) |
Black Hole Quencher 1 (534,480-580) |
0.9 |
6-FAM, fluorescein (495,520) |
Black Hole Quencher 1 (534,480-580) |
0.1 |
TAMRA (559,583) |
Black Hole Quencher 1 (534,480-580) |
We selected Black Hole Quencher 1 and 6-FAM as a quencher-fluorophore pair. Black Hole Quencher 1’s quenching range is from 480-580 nm and is well matched with 6-FAM, which has an emission maximum of 520 nm and one of the highest quantum yields, at 0.9.
Creation of a Field-deployable Fluorometer
In addition to having our system yield a fluorescent signal, we require some instrumentation that we can use to quantify this signal. We had some clear criteria for the field-based fluorometer we want to create. The fluorometer must meet the following criteria:
- Easy to use
- Portable/compact
- Affordable
- Built from commercially available parts
- Reliable
- Direct access to results
We decided to test our fluorescent signal using a cell phone fluorometer. We took inspiration from the “Lab-in-a-phone”, which is a smartphone based portable fluorometer that is used to measure pH of environmental water and other similar devises (2,3). This is an incredible system that uses an open source Android platform that is customizable to measure the desired wavelength. Inspiration from previous work done with a portable spectrophotometer for fast and reliable measurement execution is shown below (Figure 2).

Figure 2. Schematic design of a cellphone based Spectrophotmeter (3).
In part two of our project, we intend to adapt this basic design to create a cellphone based fluorometer that can be used with our system. We will construct the body of the fluorometer using plans adapted from this spectrophotometer and add excitation and emission filters to make the system ready for 6-FAM based detections. We also intend on using a cell phone app to act as the control and read out interface. We are collaborating with Team Moscow-Russia to modify an existing app, or to program a new app that would facilitate control of our fluorometer.
Prototyping and Testing a DIY Heat Block
The RPA and Flappase assays must be conducted under regulated temperatures. To provide this in the lab, we use heat blocks, but a commercial heat block would drastically increase the price of our testing system. So, we built a prototype of a heat block system using a design we found as inspiration (4).
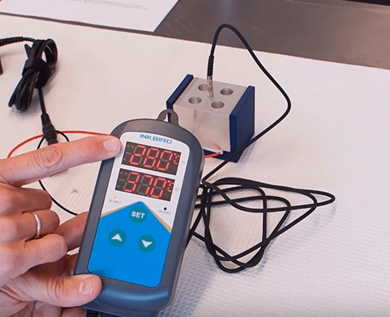
Figure 3. Heat Block Prototype. The prototype is made from common materials.
Our heat block was created out of inexpensive materials we purchased from Amazon (Figure 3). The heating/cooling device was an inexpensive Peltier chip, and the temperature was controlled by an aquarium thermostat linked to an AC adaptor. To hold the sample, we used an aluminum block into which microcentrifuge tube sized holes were drilled. A chamber to hold the aluminum block and Peltier chip was 3D printed.
We tested our DIY heat block in the lab and found that it worked fine. Materials to build the heat block were included in the Go STEM kit, and camp participants build, tested, and used their own version of the heat block for some of the activities in the “Engineering with Biology” Camp.
While the block worked fine in our hands, campers had variable results. We found than many of the Peltier chips we distributed in the kit were unreliable, and that the temperature of the heat block would fluctuate by several degrees. When assembling the kits, we tried to use the least expensive materials we could, including the Peltier chips. The design of our heat block needs further refinement, and first step in that process will be testing the system with a higher-quality heating/cooling chip.
References
- Integrated DNA Technologies - Fluorophores Modification. (n.d.). Retrieved October 25, 2020, from www.idtdna.com/site/Catalog/Modifications/Dyes
- Hossain, A., Canning, J., Ast, S., Rutledge, P. J., Yen, T. L., & Jamalipour, A. (2015). Lab-in-a-Phone: Smartphone-Based Portable Fluorometer for pH Measurements of Environmental Water. IEEE Sensors Journal, 15(9), 5095-5102. doi:10.1109/jsen.2014.2361651
- Laganovska, K., Zolotarjovs, A., Vázquez, M., Donnell, K. M., Liepins, J., Ben-Yoav, H., . . . Smits, K. (2020). Portable low-cost open-source wireless spectrophotometer for fast and reliable measurements. HardwareX,7. doi:10.1016/j.ohx.2020.e00108
- Maloney, D., Says:, E., Says:, R., Says:, W., & Says:, L. (2018, November 13). Hacked Heating Instruments For The DIY Biology Lab. Retrieved October 26, 2020, from https://hackaday.com/2018/11/12/hacked-heating-instruments-for-the-diy-biology-lab/