Development of the Flappase System
This detection system relies on the use of a 5’ Flap Endonuclease (Flappase). In this system, two DNA oligos (a spacer and a flap oligo) are designed to bind to the target DNA (Figure 1). The spacer oligo binds upstream of the SNP and has a single 3’ nucleotide complimentary to the SNP (cSNP). The Flap oligo is longer, and is composed of the following three sections:
- A 5’ flap portion, that is not complementary to the target sequence.
- A single nucleotide, complimentary to the cSNP (ccSNP).
- A 3’ targeting section, which is complementary to the target gene.
We designed two Flap oligos, one to target the A1 allele of the CSN2 gene, and one to target the A2 allele. These two Flap oligos differ only in the cSNP.

Figure 1. Design of Flap and Spacer Oligos. Spacer oligo structure with a single 3’ nucleotide cSNP (yellow). Flap oligo structure with a 5’ flap portion in light blue, ccSNP labeled orange. Dark blue region on both spacers is complementary to the target DNA.
In this system, the spacer and the flap oligo are annealed to the target DNA. In our case, we designed the oligos to be complimentary to exon 7 of the CSN2 gene. The structure created by the 3’ overhang of the spacer oligo and the 5’ flap oligo should differ, based on the SNP present in the target DNA, and the cSNP in the oligo (Figure 2).
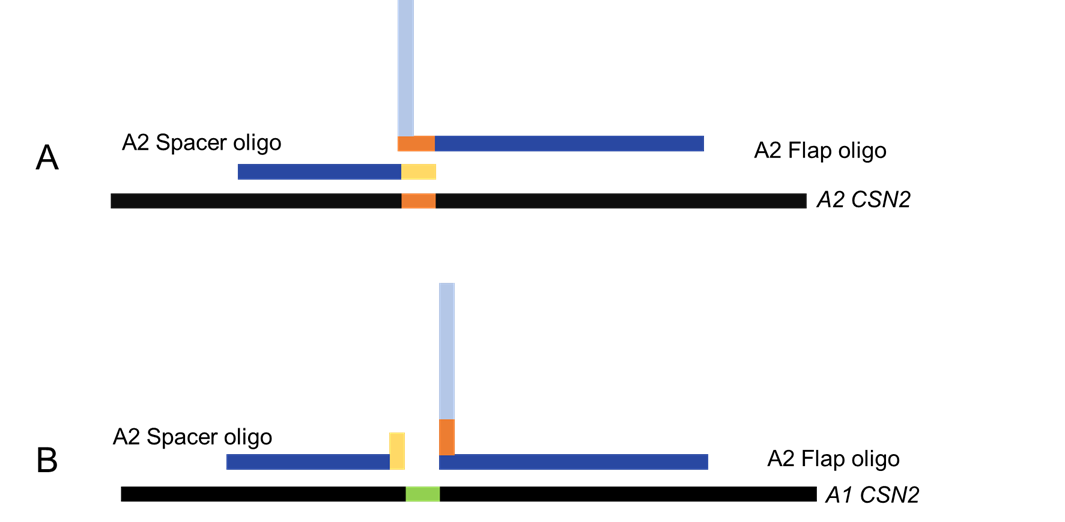
Figure 2. Annealing of the oligos to the target DNA. The successful annealing to the correct target DNA creates a Holiday-junction like structure. The junction occurs when the cSNP recognizes the SNP and binds to it. B shows the annealing of the oligos to the target DNA until an incompatible SNP, therefore not binding or crating the Holiday-junction structure.
The Flappase specifically recognizes the flap and spacer oligos when the form a Holiday-junction like structure. When that structure forms, the Flappase will cleave the Flap oligo. The 5’ and 3’ ends of the Flap oligo are tagged with a fluorophore-quencher pair. Cleavage of the flap will release the fluorophore from quenching, yielding a fluorescent signal.
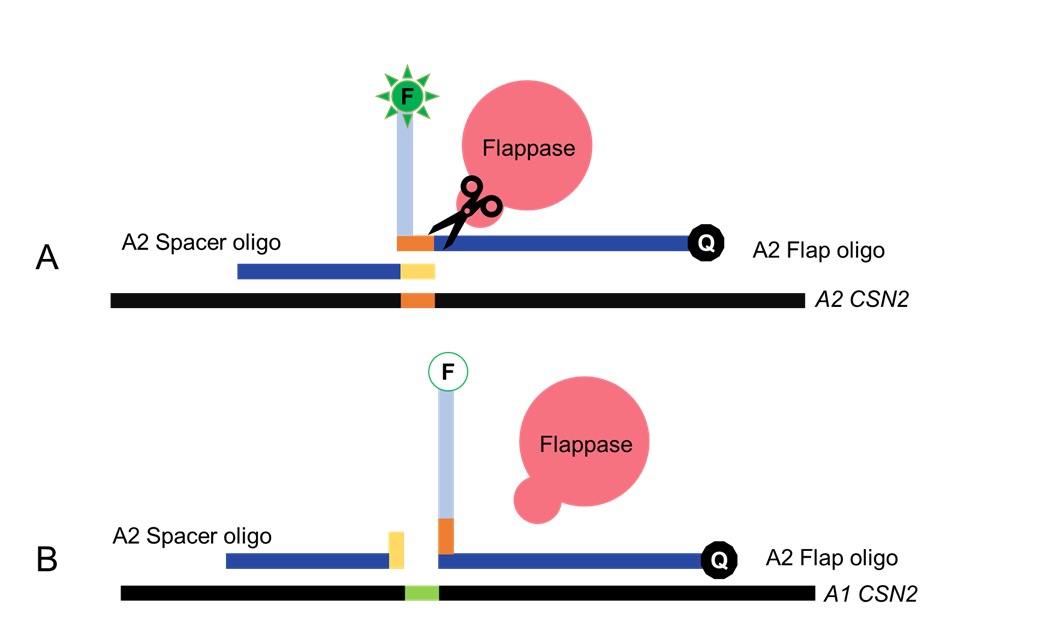
Figure 3. Recognition of Holiday junction by Flappase. The flap oligo is tagged with a fluorophore-quencher pair. A shows Flappase cleavage of the Flap oligo upon successful recognition, separating the fluorophore-quencher pair and emitting detectable light. In contrast, B shows the lack of recognition by Flappase, due to the lack of a Holiday junction. FAM fluorescence is illustrated by activation of the fluorophore (F), upon cleavage of the flap and release of FAM from Blackhole Quencher 1 (Q).
Design and Construction of the Flappase
5' Flap endonucleases are present in Bacteria, Archaea and Eukaryotes (1). Most published work on Flap endonucleases focuses on Human, Saccharomyces cerevisiae, or enzymes from the Archaeon Pyroccocus furiousus. We chose the Flappase from S. cerevisiae, also known as Rad 27 or FEN-1, as the Flappase for our system for practical reasons. Yeast grow well, and retain enzyme function, at a wider range of temperatures than Humans. Furthermore, P. Furiousus is an extremophile, growing well at temperatures above 60°C, making this heat-adapted enzyme not compatible with field testing.
We downloaded the S. cerevisiae Flappase cDNA sequence from NCBI (NCBI Reference Sequence: NM_001179679.1) and codon optimized the sequence for expression in E. coli using IDT’s codon optimization tool. We then built an expression system (Figure 4). We elected to add a 10X His tag to the C-terminal domain of the Rad27 gene, since previous studies have used this orientation with no loss of enzyme activity (2). We added a strong RBS from the iGEM toolkit, and a T7 promoter to allow us to adjust expression of the gene. We elected a tunable system, as overexpression of Flappases has been shown to have toxicity in Human and Yeast cells (3). We then cloned the part into pSB1C3.
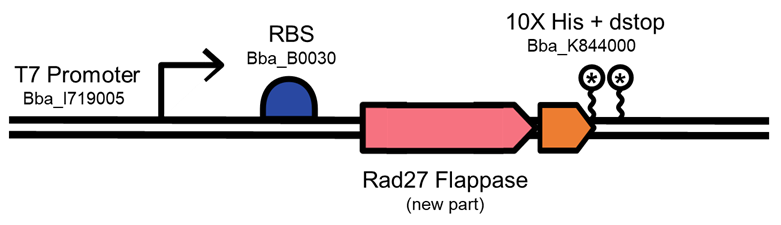
Figure 4. Expression system for Rad27 Flappase. T7 promoter and a strong RBS were added to regulate expression of Rad27, 10X His tag (for purification) and double stop codon.
We used this part to transform E. coli BL21 DE3 Cells. We verified the successful cloning of this part by colony PCR and restriction digestion (Figure 5).
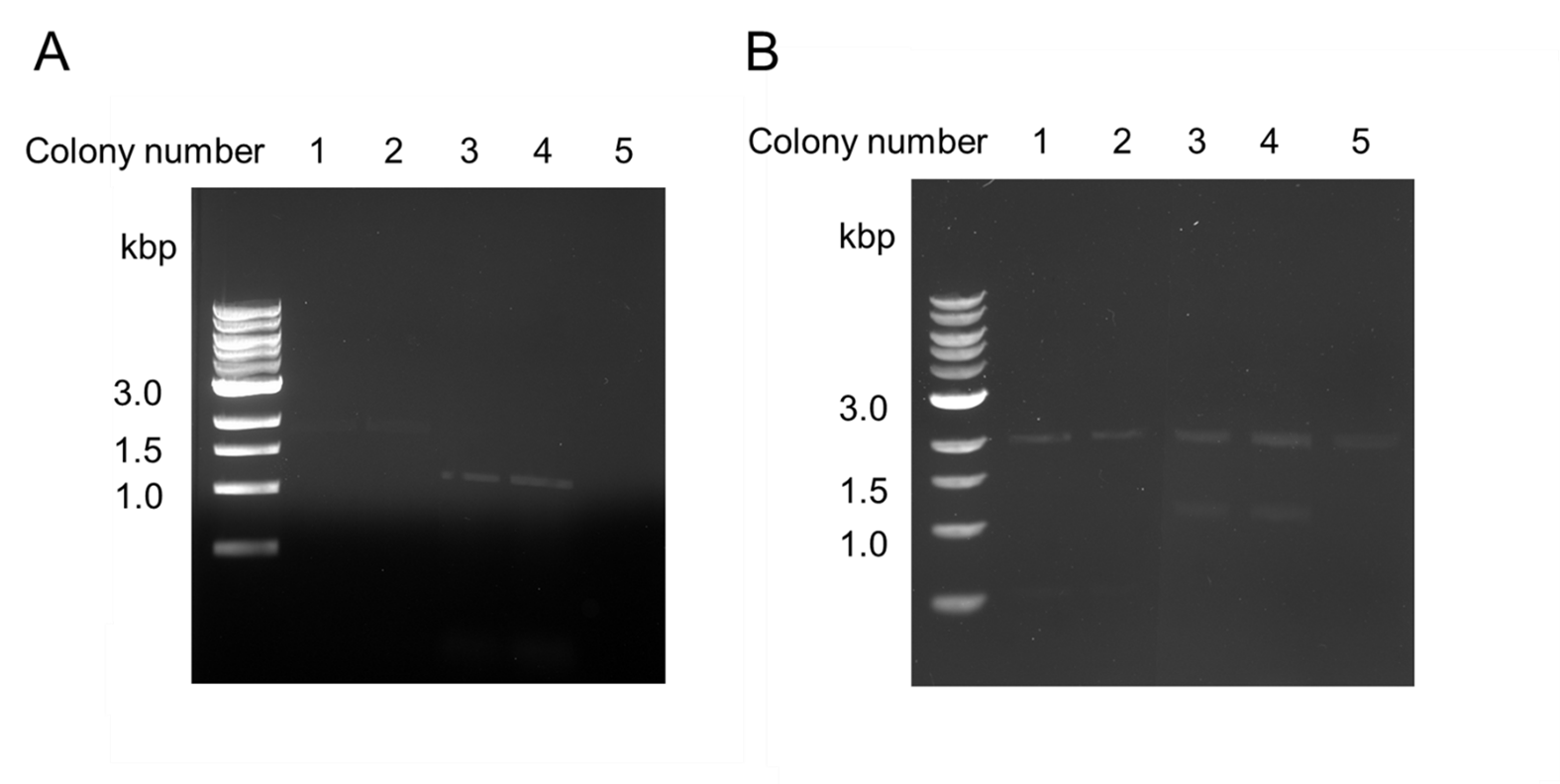
Figure 5. Verification of the Flappase expression construct. The Flappase construct was designed and ligated into pSB1C3. Ligations were then transformed into E.coli BL21. (A) Colonies were screened using colony PCR to identify clones containing the insert. (B) Minipreps and restriction digestions were used to confirm insert.
Most of the literature regarding assays using Flappase and DNA oligos used Human Flappase as the enzyme. Since we based the development of our system on work done on Human Flappase, we decided to investigate how similar the Human and S. cerevisase Flappases are, to validate the use of similar methods.
We performed a BLAST search on the amino acid sequence of these two proteins and found that S. cereviseae and human Flappase are 62% identical. Given that the two proteins are so similar, and have conserved function, we were confident that the yeast Flappse will work like human Flappase in in vitro assays using a similar oligo design. To further support this, we built a homology model for S. cerevisiae Flappase, to provide evidence that methods used with the human enzyme would be transferable to the yeast Flappase.
For information on the homology model we created, see the Modeling Page.
Design of the Flap and Spacer Oligos
We looked in the literature to determine what features our spacer and flap oligo should have. For the spacer oligo, we found that different studies used spacer oligos of different lengths (1-3). One paper reported that the presence of the single 3’ nucleotide overhang enhances recognition of the Holliday structure by the Flappase and promotes cleavage, while removal, or addition of extra 3’ nucleotides inhibits (4). Based on this, we designed an 18 nucleotides spacer oligo, with 17 nucleotides complimentary to the CSN2 gene, and one 3’ cSNP. We made one for the A1 and one for the A2 alleles of CSN2 (Figure 6).
We used the Flap oligo from Nolan et. al. as inspiration for the design of our Flap oligos (5). For the 5’ flap portion, we used the sequence from the paper directly. We then follow that section with the ccSNP to the allele of interest, and then added 18 nucleotides to the 3’ end that are complimentary to the CSN2 gene. We made one for the A1 and one for the A2 alleles of CSN2 (Figure 7).
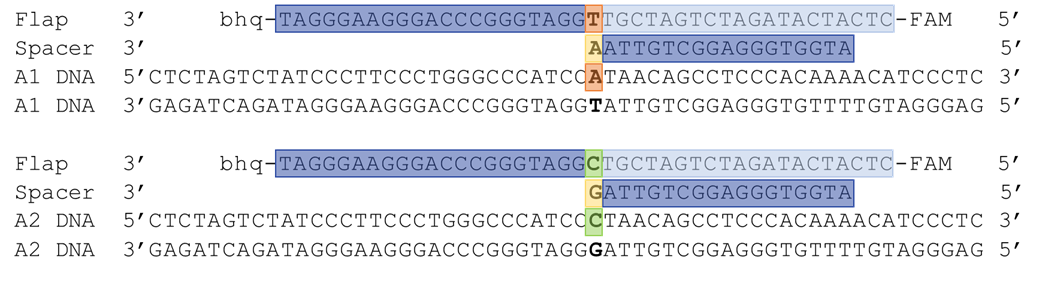
Figure 6. A1 and A2 flap and spacer oligo design. One spacer and one flap oligo were designed to target the A1 and A2 alleles of CSN2. Features of the oligos are highlighted to correspond to the different regions outlined in figure 1.
Purification of the Flappase
The next step of our project was the purification of Flapppase. We began testing procedures for the induction of expression of a His-tagged protein under the control of a T7 promoter using Tuner cells. We also, worked on optimizing lysis conditions and purification of this protein. We developed protocols for each of these steps.
Unfortunately, due to an outbreak of SARS-CoV2, our College closed all in-person activities just as we started to work on purifying the 10X-His tagged Flappase. We lost access to the lab for the remainder of this project. Purification was a key component of our project, we planned to purify Flappase by metal affinity gravity-flow column purification.
Testing the System
Once we purify the Flappase enzyme, we plan to test its activity. To do this, first, we would use the CSN2 exon 7 DNAs we have created as positive controls. We will amplify a fragment of exon 7 using the optimized RPA protocol we develop. We will then test the Flappase cleavage reaction using a reaction condition modified from previously published protocols (6-8). We intend to prepare 50 ng of RPA product (template) in TMB (50 mM Tris, 10 mM MgCl2, and 0.1 mg/mL BSA (pH 8.0) buffer. The DNA will be heated to 95 °C for 5 minutes to denature the double stranded template, and then cooled to 70 °C. 75 ng of spacer and flap oligo will be added, and the reaction cooled room temperature (20 °C) to allow annealing (flap oligos have a melting temperature of approximately 63 °C, spacer oligos approximately 53 °C). 2.5 ng of purified Flappase will be added to the reaction, and fluorescence will be measured every 2 minutes for 20 minutes to measure the kinetics of the reaction using a fluorometer. We expect that this set of reaction conditions will require extensive optimization.
References
- Hosfield, D. J., Mol, C. D., Shen, B., & Tainer, J. A. (1998). Structure of the DNA Repair and Replication Endonuclease and Exonuclease FEN-1. Cell, 95(1), 135-146. doi:10.1016/s0092-8674(00)81789-4
- Gary, R., Park, M., Nolan, J., Cornelius, H., Kozyreva, O., Tran, H., . . . Gordenin, D. (1999, August). A novel role in DNA metabolism for the binding of Fen1/Rad27 to PCNA and implications for genetic risk. Retrieved October 25, 2020, from https://www.ncbi.nlm.nih.gov/pmc/articles/PMC84380/
- Becker, J. R., Gallo, D., Leung, W., Croissant, T., Thu, Y., Nguyen, H., . . . Bielinsky, A. (2018). Flap endonuclease overexpression drives genome instability and DNA damage hypersensitivity in a PCNA-dependent manner. Nucleic Acids Research, 46(11), 5634-5650. doi:10.1093/nar/gky313
- Liu, R. (2006). The DNA-protein interaction modes of FEN-1 with gap substrates and their implication in preventing duplication mutations. Nucleic Acids Research, 34(6), 1772-1784. doi:10.1093/nar/gkl106
- Shen, B., Nolan, J., Sklar, L., & Park, M. (1997, August 15). Functional analysis of point mutations in human flap endonuclease-1 active site. Retrieved October 25, 2020, from https://www.ncbi.nlm.nih.gov/pmc/articles/PMC146887/
- Mein, C. A. (2000). Evaluation of Single Nucleotide Polymorphism Typing with Invader on PCR Amplicons and Its Automation. Genome Research, 10(3), 330- doi:10.1101/gr.10.3.330
- Yoshimi, S., Ochi, H., Murakami, E., Uchida, T., Kan, H., Akamatsu, S., . . . Chayama, K. (2015). Rapid, Sensitive, and Accurate Evaluation of Drug Resistant Mutant (NS5A-Y93H) Strain Frequency in Genotype 1b HCV by Invader Assay. Plos One, 10(6). doi:10.1371/journal.pone.0130022
- Nolan, J. P., Shen, B., Park, M. S., & Sklar, L. A. (1996, September 10). Kinetic Analysis of Human Flap Endonuclease-1 by Flow Cytometry. Biochemistry, 35(36), 11668–11676. https://doi.org/10.1021/bi952840